The bone of contention in the evolution of vertebrates
Departments Research impact and institutes 2 August 2018
It takes backbone to try and tackle one of the age-old mysteries of the evolution of life, but a researcher at The University of Manchester has proven he’s certainly not too spineless to take on the challenge.
Just like close to ten per cent of all life on Earth, we humans are vertebrates – meaning we have a backbone that protects our spinal cord. Nearly all vertebrates living today fall into the Gnathostomata group – we’re jawed vertebrates.
Fishy ancestors
Gnathostomata evolved some 400 million years ago, when life on Earth greatly diversified during what is known as the Cambrian Explosion. Among this menagerie was a group of fishes called heterostracans (of which the anglaspis, pictured above, was one), which included some of our earliest vertebrate ancestors to have left behind a mineralised skeleton.
The earliest vertebrates had un-mineralised skeletons, and because un-mineralised tissue is more at risk of decay, its fossil record has been patchy. Heterostracans, therefore, provide scientists with one of the earliest examples of a vertebrate skeleton – and an understanding of how this evolved and diversified.
The aspidin conundrum
More than150 years ago, comparative anatomist Thomas Henry Huxley put a heterostracan skeleton under the microscope to take a closer look at the tissue from which it was made. This tissue is named ‘aspidin’, but since its first examination, there has been some controversy over how exactly it should be classed.
There are four types of tissue found in present-day skeletons: dentine and enamel, which teeth are made from, and bone and cartilage, which forms our skeleton. Some scientists have argued aspidin was a transitional tissue – an example from an early stage in the evolution of bone – rather than true bone, as we know it today.
One reason for this confusion is that early studies discovered a network of tubes within the aspidin tissue. Such tubes are not a component of modern mineralised tissue, leading many to assume that aspidin was a transitional tissue. Everything from cracks during fossilisation to cell spaces for bone or dentine were suggested as the causes of the tubes.
Then, in the mid-20th Century, 2-dimentional sectioning showed that the tubes appeared to branch out. This process is seen in bone and dentine tissue – but, if anything, the discovery just caused more confusion, rather than solving the mystery.
A closer look
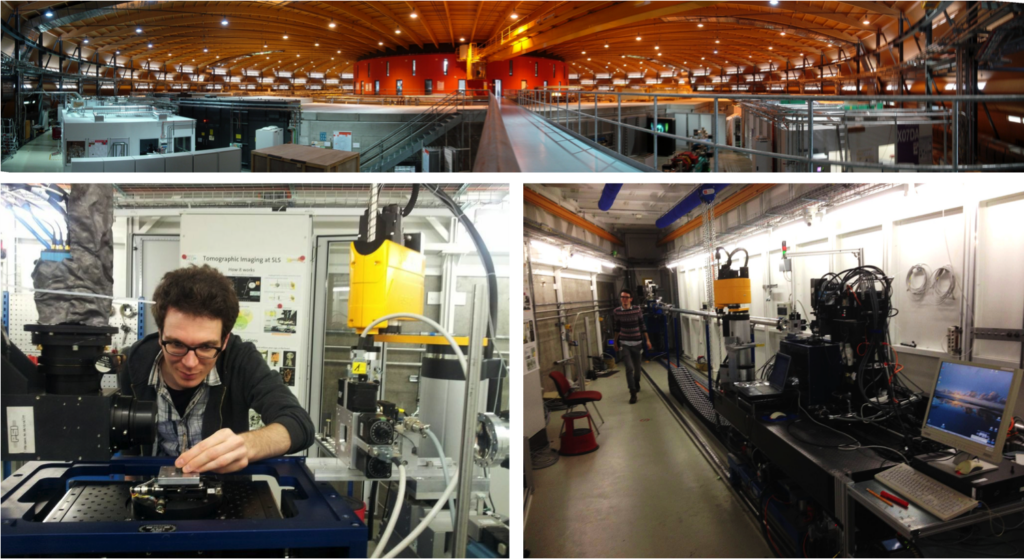
Now, Dr Joseph Keating, of the School of Earth and Environmental Sciences, hopes he’s answered the question once and for all. As Lead Researcher on a project that includes scientists from The University of Manchester and the University of Bristol, Dr Keating used a powerful type of CT scanning to take a closer look at aspidin than has ever been achieved before.
Synchrotron tomography uses a particle accelerator to generate high energy x-rays of a single wavelength to scan objects. These scans allowed the team to create a 3D digital model of aspidin – and in so doing they realised there was something fishy about those ‘branching’ tubes.
In fact, they didn’t branch at all. Dr Keating notes that this misunderstanding arose from the appearance of the tubes in earlier 2D sectional images. The latest models, published in the journal Nature Ecology and Evolution, show the tubes lack any branching, which means they weren’t dentine or bone cell spaces.
Rather, the spaces are likely to have housed bundles of collagen fibres. Collagen is present in bone and most mineralised tissues and acts as the scaffold around which the minerals are deposited. This led the team to conclude that, rather than a transitional material, aspidin provides the earliest example of bone in the fossil record.
Reassessing the fossil record
Co-author Professor Phil Donoghue from the University of Bristol explains: “These findings change our view on the evolution of the skeleton. Aspidin was once thought to be the precursor of vertebrate mineralised tissues. We show that it is, in fact, acellular dermal bone, and that all these tissues must have evolved millions of years earlier [than once thought].”
While one mystery has been solved, the discovery raises new questions. Dr Keating notes in his blog about the findings that the results suggest all mineralised tissue appeared “simultaneously in the fossil record”, during the Cambrian Period 420 million years ago. This means we don’t yet know when the tissue first evolved, or why the examples all appeared at the same time.
One possible answer to the second question is that un-mineralised early bone tissue has not left behind a record. Dr Keating hypothesises that bone and dentine evolved as layers of skin that predated the evolution of the genetic pathways which make mineralisation possible.
Dr Keating concludes: “It is entirely possible that future fossil discoveries will reveal even earlier stages in the evolution of the skeleton, allowing us to solve these new mysteries. Of course, these fossils may have already been discovered and are currently sitting in museum drawers, awaiting study. Perhaps it is time to revisit the skeletons in our closets.”
Words – Hayley Cox
Images – The University of Manchester and Dr Joseph Keating